A new solar project was just installed in the US.
Set a timer for 60 seconds and wait. Maybe take a step outside and soak up some sunlight.
Zing!—another solar project was just installed.
The sun is shining on the solar industry; the numbers are impressive. Today, there is enough solar capacity in the US to power the equivalent of 23 million homes, according to the Solar Energy Industries Association (SEIA). That’s 126 gigawatts (GW), coming from millions of solar systems across the country.
The US solar industry was valued at $33 billion in 2021, employed more than 230,000 people, and continued to grow in power capacity at an average rate of 33 percent per year.
Solar panels generated almost 4 percent of electricity in the US in 2021, up from less than 1 percent in 2015. In some places that number is much higher; for example, 17% of California’s electricity generation came from solar in 2021. Almost half of all new energy capacity added to the US grid in 2021 came from solar. Even more encouraging, by 2030, the solar industry aims to generate nearly a third of US electricity.
With so many solar panels planned for the coming years, you might be wondering: what exactly are solar panels and how are they made?
Meet your solar panel
There are two types of solar technology for electricity generation. The most common are photovoltaic (PV) panels or modules, which use the sun’s light to make electricity. Another technology, concentrating solar power (CSP), uses the sun’s heat instead.
The most common type of PV panel is made using crystalline-silicon (c-SI). That technology accounts for 84% of US solar panels, according to the US Department of Energy. Other types include cadmium telluride, copper indium gallium (di)selenide panels, and thin-film amorphous silicon. Because c-SI panels compose most of the US and global market, I focus on them in this blog.
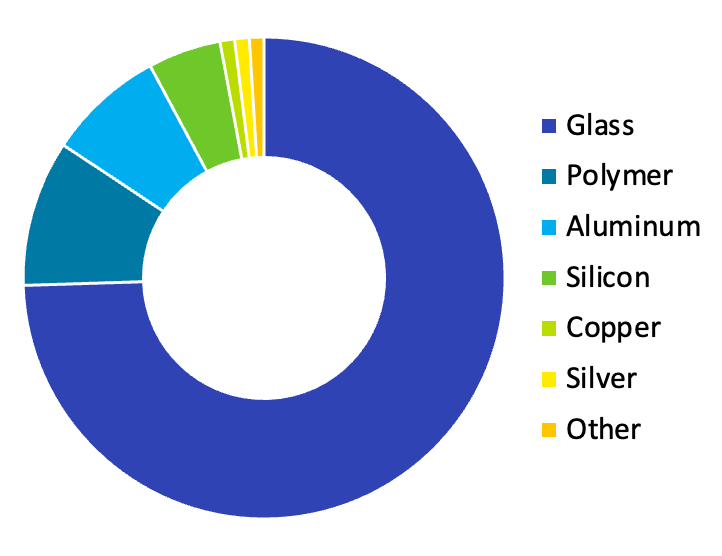
Building a crystalline silicon solar panel is a bit like building a sand castle, because silicon comes from sand! Beach sand is silicon dioxide, aka silica. (If beach patrol put that on a warning sign, I bet no one would step foot on the beach!). Silicon, in the form of silicon dioxide sand and gravel, is the second most abundant element on Earth, next to oxygen.
Before it’s used in a solar panel, silicon dioxide must be turned into pure “metallurgical grade silicon” (MGS). This process uses a lot of energy: producing 1 kilogram of metallurgical grade silicon requires 14-16 kWh of power, which is roughly equivalent to using your home oven for seven hours. Still, over their lifetimes, solar panels emit 25 times less carbon dioxide equivalent per kilowatt hour than coal-powered electricity.
Chemistry break! The recipe for cooking up metallurgical grade silicon is
Add 1 part silicon dioxide (gravel) and 2 parts carbon (sourced from coal, charcoal, or wood chips) to an electric arc furnace
Crank up the heat to 2200 degrees Celsius (this is a third of the temperature of the sun!!)
Ta-da! You’re left with 99% pure silicon and carbon monoxide (that’s from the carbon we added, bonded to the oxygen we removed from the silicon dioxide)
But solar panels are perfectionists; they demand silicon to be close to 100% purity. To achieve that, we need to upgrade the silicon into an even more pure polysilicon metal using a process that involves hydrochloric acid and hydrogen gas. (Fun fact: about 12% of the world’s silicon production is currently processed into polysilicon for solar panels.)
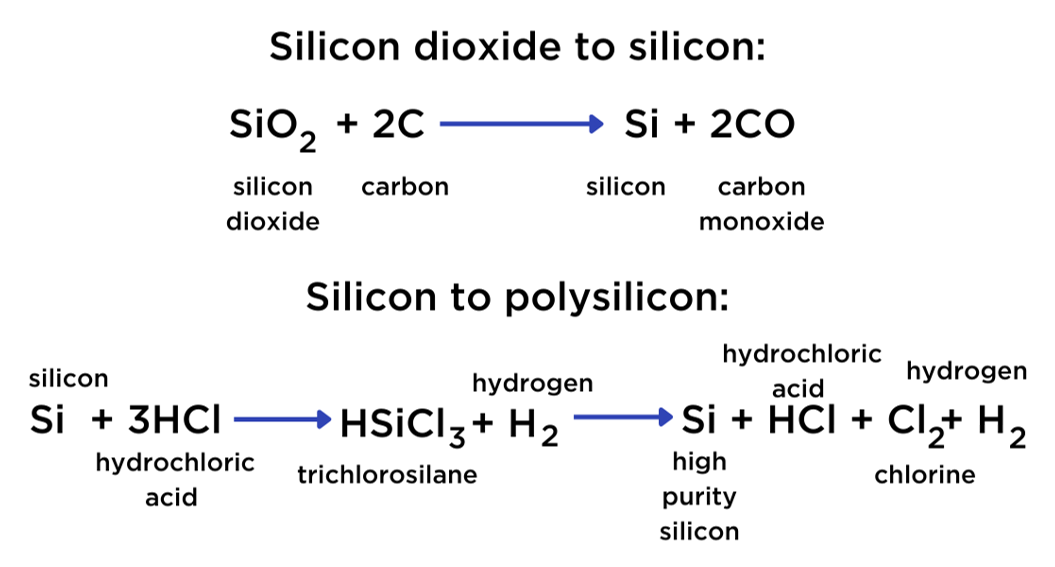
From sand to modules
After adding the acid and gas, we are left with chunks of polysilicon metal, which are typically melted down again in a roughly 5-meter-long cylindrical mold. Boron is added to give the metal a positive electric charge on one side. The hot, melty silicon cools and forms a single crystal (“monocrystalline”) structure as a cylindrical ingot. Ingots are any material cast into a rectangular shape, like bars of gold.
(Another process is used to make “polycrystalline” silicon wafers, in which multiple crystals form. This process tends to lead to less efficient panels but can reduce the cost of wafers.)
Next, a wire saw cuts the pure metal blocks of polysilicon into paper-thin, typically 7-inch by 7-inch flat slices called wafers.
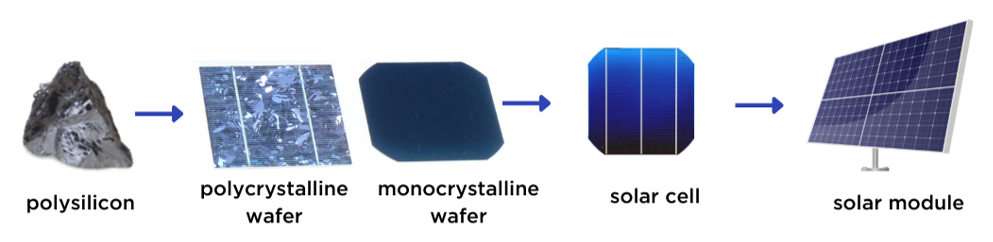
The wafers are heated in an oven and a thin layer of phosphorous is added, giving one side (the opposite of the positive boron side) a negative charge. Next, an anti-reflective coating is added to the wafers because without it these shiny disks reflect sunlight—and we want them to absorb it instead. At this stage, the wafers are now capable of absorbing the sun’s energy and converting it into electrons. Now we need to add silver metal conductors so those electrons can get turned into an electrical current that devices can use!
Silver—the most conductive element in the world—intercepts the electrons in the silicon wafers and turns them into current. The silicon wafers now form a conductive solar cell. Each solar panel, usually containing 60 or 72 cells, uses about 20 grams of silver—a fraction of the panel’s weight but about 10% of its total cost.
Copper metal conductors and wiring connect the solar cells together into one big solar panel, giving it the classic matrix appearance. Copper is a good electrical conductor and very malleable, making it a great material for forming the wiring that moves the current through the panel.
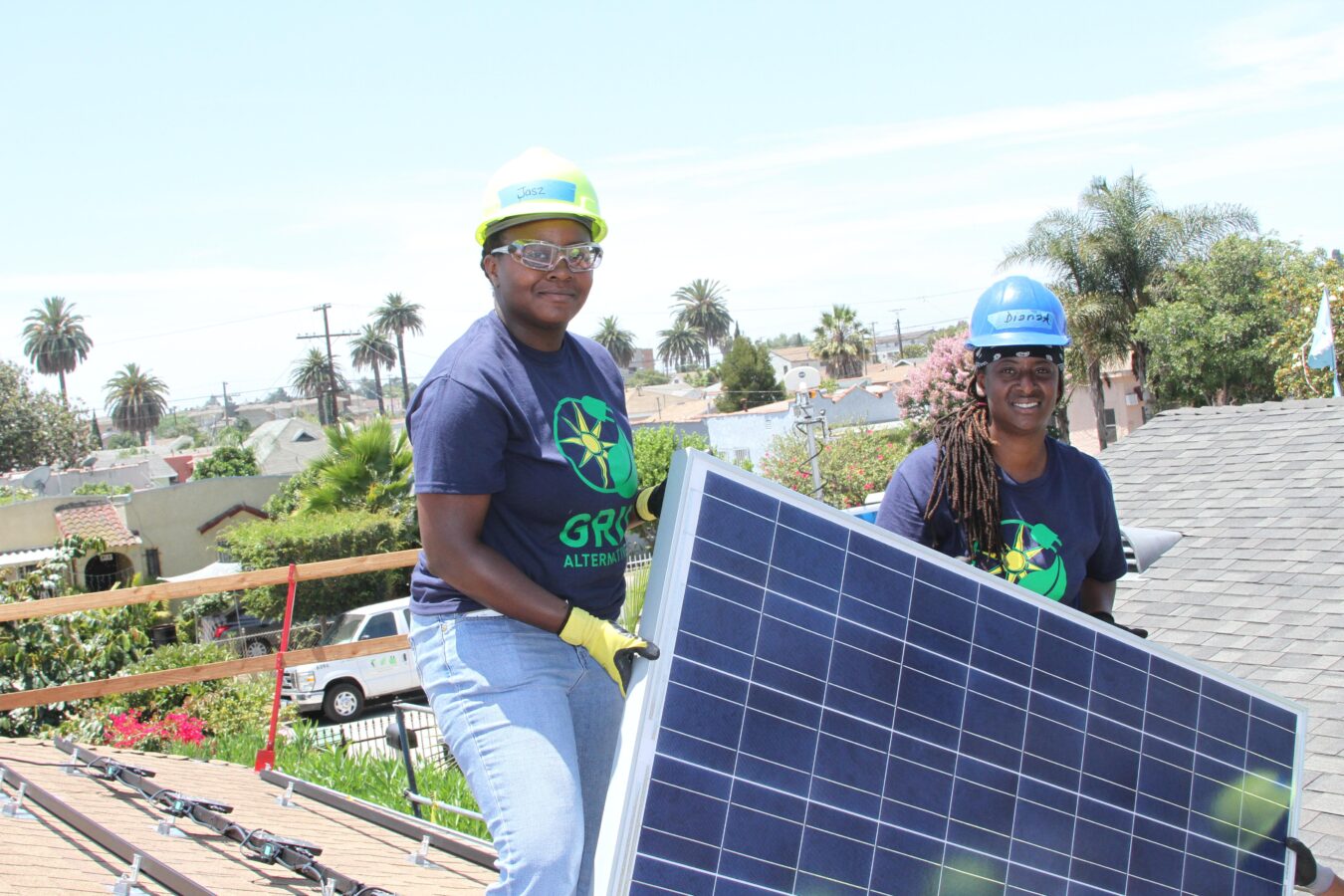
Zap! A solar panel has been made.
Now multiply it by about 60 million for the US alone, each year.
And then speed it up because we need solar to play an ever-growing role in achieving our clean energy and climate goals.
There’s a lot that goes into making solar happen beyond building panels, but responsibly accelerating solar panel manufacturing and installation is a critical step in the journey towards a just, sustainable renewable energy future.
Want to learn more about the solar panel supply chain? Which countries are driving PV material mining and PV manufacturing? What does the solar panel repair and reuse industry look like today? How can we recycle solar panel materials and create a lower-waste circular supply chain? Click the links for answers!
Mining Raw Materials for Solar Panels: Problems and Solutions